Zeolites are microporous crystalline solids that possess a primary
structure composed of MOn polyhedra,
where M is usually silicon or aluminum. The most frequently encountered
polyhedra in zeolites are tetrahedra (n=4) and they are arranged such
that each oxygen atom on one tetrahedron is attached to the central
atom of another. The tetrahedra combine in an ordered fashion to form
polygonal or polyhedral units, which in turn combine to form the framework
structure of zeolites. The progression from a single tetrahedron all
the way to the framework structure of the faujasite class of zeolites
is shown below...
Imagine taking a bottle so small that only one or two molecules
will fit inside of it! The rightmost structure in the figure above
is known as a supercage, and it is just large enough (diameter
= 1.3 nm) to fit one or two small to medium-sized organic molecules.
It turns out that molecules can often undergo completely different
reactions inside these zeolites than they do in solution (the traditional
way of running reactions). This is a good thing because we can
get molecules to react in ways that were never believed possible.
For example, a molecule (e.g. a potential drug) that may take 6-8
steps to make using traditional methods might take only 1-3 steps
to make when zeolites are employed. Another way that we use zeolites
is to cause reactions to proceed by what is normally the least
favorable pathway in solution. We are also interested in investigating
how molecules behave inside zeolites. Finding new reactions that
take place inside zeolites and the study of zeolite properties
is currently a hot topic in chemistry and there are many projects
within my research group through which you can contribute.
Papers in this area (student coauthors underlined)
J. Sivaguru, H. Saito, M. R. Solomon, L. S. Kaanumalle, S. Lakshmi,
T. Poon, S. Jockusch, W. Adam, V. Ramamurthy, Y. Inoue,
and N. J. Turro “Control of Chirality by Cations in Confined
Spaces: Photooxidation of Enecarbamates Inside Zeolite
Supercages” Photochemistry and
Photobiology, 2006, vol 82, pp 123-131.
J. Sivaguru, T. Poon, R. Franz, S. Jockusch, W. Adam, and N. J.
Turro “Stereocontrol Inside Confined Spaces: Enantioselective
Photooxidation of Enecarbamates Inside Zeolite Supercages” Journal of the American Chemical Society, 2004, vol 126, pp
10816-10817.
T. Poon, N. J. Turro, J. Chapman, P. Lakshminarasimhan, X. Lei,
W. Adam, and S. Bosio “A Supramolecular ‘Ship in Bottle’ Strategy
for Enantiomeric Selectivity in Geminate Radical Pair
Recombination” Organic
Letters , 2003, vol 5, pp 2025-2028.
|
Molecular oxygen (O2) is everpresent
in our everyday lives. It is in the air we breath and in our bodies
and that of other living organisms. Oxygen exerts both beneficial
as well as detrimental effects on nearly everything that it encounters.
For example, it is responsible for the spoilage of food on the one
hand, but on the other hand, the lack of oxygen is responsible for the
pain we feel in our muscles upon excessive physical exertion. Because
oxygen and its chemistry are so prevalent, it is important that we
understand as much as possible about its reactivity.
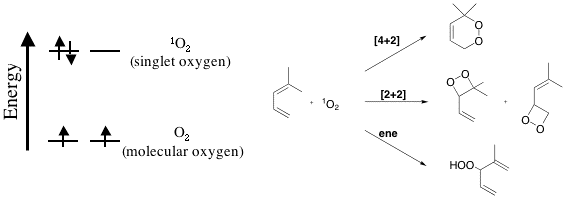
Singlet oxygen is a form of oxygen that occurs when oxygen has
been excited to a higher energy level. It has been
implicated in biological damage in several studies
and has also been used as
a reagent for a variety of organic reactions. The electronic
configuration of singlet oxygen and ground state oxygen
(molecular oxygen that
we breath everyday) is shown in the figure above. Also
shown above are examples of reactions that singlet
oxygen undergoes. In this
group, we are interested in understanding the factors
that affect the reactivity of singlet oxygen. Such
factors include solvent,
temperature, the structure of the compound that 1O2 is
reacting with, pressure, etc. The goal of these projects
is to study the reactions of 1O2 in
model systems, especially those pertinent to biology
or biochemistry, and to discover how the factors mentioned
above contribute to their
overall reactivity.
Papers in this area (student coauthors underlined)
J. Sivaguru, M.R. Solomon, T. Poon, S. Jockusch, S.G. Bosio, W. Adam, and N. J. Turro. “The Reaction of Singlet Oxygen with Enecarbamates: A Mechanistic Playground for Investigating Chemoselectivity, Stereoselectivity and Vibratioselectivity of Photooxidations” Accounts of Chemical Research (in press).
J. Sivaguru, T. Poon, C. Hooper, H. Saito, M. Solomon, S. Jockusch,
W. Adam, Y. Inoue, and N. J. Turro. “A Comparative Mechanistic
Analysis of the Stereoselectivity Trends Observed in
the Oxidation of Chiral Oxazolidinone-Functionalized
Enecarbamates by Singlet
Oxygen, Ozone and Triazolinedione” Tetrahedron (Symposia-in-Print;
invited review article), 2006, vol 62, pp 10647-10659.
J. Sivaguru, M.
R. Solomon, H. Saito, T. Poon, S. Jockusch, W. Adam,
Y. Inoue, and N. J. Turro “Conformationally controlled
(entropy effects), stereoselective vibrational quenching
of singlet oxygen in the oxidative cleavage of oxazolidinone-functionalized
enecarbamates through solvent and temperature variations” Tetrahedron (Symposia-in-Print;
invited review article), 2006, vol
62, pp 6707-6717.
J.
Sivaguru, H. Saito, T. Poon, T. Omonuwa, R. Franz,
S. Jockusch, C. Hooper, Y. Inoue, W. Adam, and N. J. Turro “Stereoselective
Photooxidation of Enecarbamates: Reactivity of Ozone
vs Singlet Oxygen” Organic Letters, 2005, vol
7, pp 2089-2092.
T. Poon, J. Sivaguru, R. Franz, S. Jockusch, C. Martinez, I. Washington,
W. Adam, Y. Inoue, and N. J. Turro "Multidimensional Control
of Stereoselectivity in the Reactions of Singlet Oxygen
with Oxazolidinone-substituted Enecarbamates" Journal
of the American Chemical Society, 2004, vol 126,
pp 10498-10499.
T. Poon, N. J. Turro, J. Chapman, P. Lakshminarasimhan, X. Lei,
S. Jockusch, R. Franz, W. Adam, and S. Bosio “Stereochemical
Features of the Physical and Chemical Interactions of Singlet Oxygen
with Enecarbamates” Organic Letters, 2003, vol
5 , pp 4951-4953.
|
Liquidambar styraciflua, commonly known as the sweetgum tree, is characterized by its mace-like fruit which falls from the tree after the fruit has released its seeds. The tree is one of the few that possesses leaves that change color in warm weather climates. Its ornamental nature is the reason that sweetgum has been planted in many municipalities throughout the United States. Our research group was the first to discover that an important chemical, shikimic acid, can be obtained in relatively large quantities from its seeds. Shikimic acid is the key ingredient in the industrial synthesis of oseltamivir, the only drug capable of treating the H5N1 form of the avian flu.

Claremont, CA is known as the "City of Trees and PhD's" because of its abundance of flora and residents with graduate degrees per capita. We are currently exploring new ways to utilize the natural products found within the vast variety of plant organisms found in the city.
Papers in this area (student coauthors underlined)
L. B. Enrich, M. L. Scheuermann, A. Mohadjer, K. R. Matthias, C. F. Eller, M. S. Newman, M. Fujinaka, and T. Poon, "Liquidambar styraciflua: a renewable source of shikimic acid" Tetrahedron Letters 2008, 49, 2503-2505.
Listen to Dr. Poon's National Public Radio (NPR) interview on this discovery. |